Table of Contents
What is Ohm’s law?
Ohm’s law is a fundamental principle in electrical engineering and physics that describes the relationship between voltage, current, and resistance in an electrical circuit. It was named after the German physicist Georg Simon Ohm, who first formulated this law.
Ohm’s law states that the current () flowing through a conductor between two points is directly proportional to the voltage (V) across the two points, while the constant of proportionality is the resistance () of the conductor. Mathematically, Ohm’s law can be expressed as:
V=I×R
where:
- is the voltage across the conductor,
- is the current flowing through the conductor, and
- is the resistance of the conductor.
This relationship implies that if the voltage across a conductor is increased, the current through it will also increase, assuming the resistance remains constant. Similarly, if the resistance of the conductor increases, the current will decrease for a constant voltage.
Ohm’s law forms the basis for understanding the behavior of various electrical components and circuits, and it is a fundamental principle used in the analysis and design of electrical systems.
Ohm’s law formula
Ohm’s formula is a very basic electrical formula. All electricians and electrical engineers should study and understand it. The formula is listed below.
Current (I) = Voltage (V) / Resistance (R)
Similarly, we can simplify it
I = V/R
While I is the current of the circuit in ampere, V is the voltage in volts and R is the resistance in Ohm.
Using Ohm’s Law, we can calculate the value of Voltage and Current. The formula for Voltage Calculation: V = I * R
Where Voltage (V) is In Volts, Current (I) in Ampere, and Resistance (R) in Ω
The formula for Resistance Calculation:
R = V/I
Hence from the equation given above, if any two values i.e. Current, Voltage, or Resistance are given. We can find the unknown value Using this Law. To find a simple relation between these values we Use Ohm’s Law Triangle.
Ohms Law Triangle
If we transpose a standard equation of Ohm law using a triangle. It gives us a combination of different values.
This triangle is used to help in memorizing the 3 forms of the law. The main triangle looks like the below one.
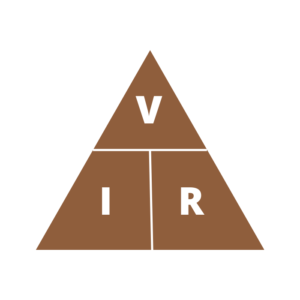
For finding different combinations we can use the triangle to simplify the formulas. As we discussed above electrical circuits are made of devices. So these devices can be divided into two different categories.
ohm’s law examples
Assume we have an electrical circuit. In this circuit, we have a battery of 12 V and a load of 6 Ω resistance. Calculate the current which should pass through the load.
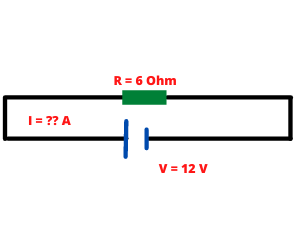
Using Ohm’s law formula we can find the current as follows: I = V/R = 12 / 6 = 2 A Now the same law is applicable to find the value of both resistance and voltage.
Assume the same circuit with the same voltage and the current passing through the load is 2 A. We need to calculate the value of the load resistance.
Using the same Ohm’s formula we can find the resistance as follows: R = V/I = 12 / 2 = 6 Ω Assume the same circuit with the same resistance and the current passing through the load is 2 A. We need to calculate the value of the battery voltage.
Using the same Ohm’s formula we can find the voltage as follows: V = I*R = 2*6 = 12 V In this way, we calculated all parameters of the electrical circuit simply by using Ohm’s law formula.
Is Ohm’s law applicable to both DC and AC circuits?
Ohm’s law is applicable to both DC (direct current) and AC (alternating current) circuits but with some considerations for AC circuits.
In the case of DC circuits, where the current flows in one direction, Ohm’s law holds true in its simplest form. The voltage, current, and resistance are constant and can be directly calculated using the formula:
V=I×R
However, in the case of AC circuits, where the current periodically changes direction, Ohm’s law is applicable under certain conditions.
In AC circuits, the concept of impedance (), which is similar to resistance but considers the effects of both resistance and reactance (due to inductance and capacitance), is used. The relationship between voltage, current, and impedance in an AC circuit is described by the following equation:
V=I×Z
where:
- V is the voltage across the circuit,
- I is the current flowing through the circuit, and
- is the impedance of the circuit, which includes both resistance and reactance.
Impedance can be more complex than resistance, as it depends not only on the resistance of the circuit but also on the frequency of the AC signal and the reactive components present in the circuit.
In AC circuits, the phase relationship between voltage and current also becomes a factor, and the concept of impedance triangle and phasors is used to represent the relationship between voltage, current, and impedance.
In summary, Ohm’s law is applicable to both DC and AC circuits, but for AC circuits, the concept of impedance is used to incorporate the effects of both resistance and reactance.
Why does voltage increase when the current decreases?
In a simple electrical circuit, if the resistance remains constant and the power source (such as a battery) remains the same, a decrease in current is typically associated with an increase in voltage. This relationship can be explained by Ohm’s law and the conservation of energy.
According to Ohm’s law:
V=I×R
where:
- is the voltage,
- is the current, and
- is the resistance.
If the resistance remains constant (R is fixed) and the current (I) decreases, the voltage () across the circuit will increase proportionally to maintain the equation’s balance. This implies that as the current decreases, the voltage has to increase to maintain the same power output.
In practical applications, this relationship is often observed when a variable load is connected to a constant voltage source.
As the load resistance increases, the current flowing through the circuit decreases, and the voltage across the load increases accordingly.
This effect is commonly seen in electrical systems and can be utilized in various applications, such as in voltage regulation and power management.
Why do some devices not obey Ohm’s law?
There could be several reasons for a device not following Ohm’s law. Devices that do not obey Ohm’s law are called non-ohmic devices.
A common reason for devices not following Ohm’s law is that they are non-ohmic devices. Some devices do not show linear behavior in their V-I graph.
For example, in the filament lamp, the resistance changes dramatically. The resistance of the filament lamp is very low at the start but when there has been some time passed since it started, the resistance increases too much.
At the start, the resistance has a smaller value than the normal value because the temperature of the filament bulb is too low. At lower temperatures, the electron collision is very low therefore resistance is also low.
As we know the temperature of the filament lamp increases with time, and so does the resistance. All of this behavior plots a non-linear V-I graph. Other than the non-ohmic device, we also call the filament lamp a non-linear device.
Other examples of non-linear devices are diodes and thermistors. There are 2 reasons for a thermistor to be non-ohmic: positive temperature coefficient thermistor and negative coefficient thermistor.
In a positive coefficient thermistor, the resistance increases as the temperature increases. But in the negative coefficient, the resistance decreases, as the temperature increases.
However, according to Ohm’s law, the resistance must not be affected by the temperature. That’s why it is a non-ohmic device.
Is Ohm’s law applicable to high voltage?
Ohm’s law is applicable to high voltage systems, provided that the basic assumptions underlying the law remain valid.
These assumptions include the linearity of the circuit components and the constancy of the material’s resistance with changes in voltage and current.
However, when dealing with high voltage systems, engineers and electricians must also consider various factors and challenges that arise at these voltage levels, including:
-
Insulation Requirements: High voltage systems require special insulation materials and techniques to prevent electrical breakdown and ensure safety. Insulators need to be carefully selected to withstand the high electric field strength associated with high voltages.
-
Corona Effects: At high voltages, there is a risk of corona discharge, which can lead to power loss, radio interference, and even damage to the equipment. Engineers must consider the effects of corona and take appropriate measures to minimize its impact.
-
Safety Considerations: Working with high voltages presents significant safety risks. Proper safety protocols, equipment, and training are crucial to ensure the safety of personnel working with or around high voltage systems.
-
Transmission Losses: High voltage transmission systems often experience higher resistive losses and corona losses, leading to reduced efficiency. Engineers must carefully design transmission systems to minimize these losses and ensure efficient power transmission.
While Ohm’s law remains a fundamental principle for understanding the behavior of electrical circuits, working with high voltage systems requires a thorough understanding of the additional complexities and challenges associated with these systems.
Engineers and electricians must apply advanced principles and specialized knowledge to ensure the safe and efficient operation of high voltage systems.
When a bulb is turned ON, is Ohm’s law applicable?
Yes, Ohm’s law is applicable when a bulb is turned on. Ohm’s law states that the current flowing through a conductor between two points is directly proportional to the voltage across the two points. This relationship is characterized by the equation:
V=I×R
Where:
- V: is the voltage across the conductor,
- I: the current flowing through the conductor, and
- is the resistance of the conductor.
When a bulb is turned on, it acts as a resistor, and Ohm’s law describes the relationship between the voltage applied across the terminals of the bulb, the current flowing through the bulb, and the resistance of the bulb.
The resistance of a bulb can change slightly with temperature, but for practical purposes, Ohm’s law can be used to describe the behavior of the bulb when it is turned on.
Does Ohm’s law hold for liquid electrolytes?
Ohm’s law can be applicable to certain liquid electrolytes, specifically those that behave as ohmic conductors. Ohmic conductors are materials that obey Ohm’s law, where the current through the conductor is directly proportional to the voltage applied across it.
However, it’s important to note that not all liquid electrolytes follow Ohm’s law precisely. The behavior of electrolytes can be more complex due to factors such as concentration, temperature, and the presence of ions. Some electrolytes may show non-linear behavior due to electrochemical reactions at the electrodes or other factors such as ion migration, which can cause deviations from Ohm’s law.
In cases where the electrolyte behaves non-linearly, the relationship between voltage, current, and resistance may not be accurately described by Ohm’s law. Instead, more complex models such as the Nernst equation or the Goldman-Hodgkin-Katz equation might be necessary to describe the behavior of electrolytes accurately.
Therefore, while Ohm’s law can serve as a basic principle, the behavior of liquid electrolytes is often more intricate, and their conductivity may depend on various factors beyond just the applied voltage.
Is Ohm’s law applicable to copper wire?
Yes, Ohm’s law is applicable to a copper wire, assuming the temperature remains constant and the wire is within its elastic limit.
A copper wire, in its normal operating conditions, can be considered an ohmic conductor. This means that its resistance remains approximately constant for a given temperature and can be described by Ohm’s law.
Ohm’s law states that the current flowing through a conductor is directly proportional to the voltage across the two points of the conductor, given that the temperature remains constant. This relationship is characterized by the equation:
V=I×R
Where:
- is the voltage across the conductor,
- I is the current flowing through the conductor, and
- is the resistance of the conductor.
In the case of a copper wire, the resistance (R) can be considered constant under normal conditions.
Hence, if you increase the voltage applied across the ends of the copper wire, the current flowing through it will increase in proportion, following Ohm’s law.
Similarly, if the resistance of the wire changes due to factors such as temperature, then Ohm’s law might not be directly applicable, and more complex models would be required to describe its behavior accurately.
Is Ohm’s law applicable to a half-wave rectifier?
Ohm’s law can be applicable to a half-wave rectifier circuit, with some considerations for the characteristics of the diode used in the rectifier.
In a half-wave rectifier, the diode conducts current only during the positive half-cycle of the input AC signal, blocking the negative half-cycle. The rectified output is a pulsating DC waveform with a significant ripple.
While Ohm’s law is not directly applicable to the diode itself due to its non-linear characteristics, it can be used to analyze the behavior of the resistor or load connected in the circuit.
Ohm’s law helps determine the current through and voltage across the resistor or load, considering the average or peak values of the rectified waveform.
However, the analysis of the rectifier circuit as a whole would require a more comprehensive understanding of the characteristics of the diode, the load resistance, and the interaction between the components.
In practical scenarios, circuit analysis for rectifiers often involves the consideration of diode characteristics, including forward voltage drop, reverse recovery time, and dynamic resistance, in addition to the principles of Ohm’s law.
This comprehensive analysis helps in understanding the behavior of the rectifier circuit and the output waveform more accurately.
Does our body obey Ohm’s law?
The human body’s behavior in response to electric current is not strictly in accordance with Ohm’s law, primarily due to the complex nature of the body’s tissues and the varied pathways that current can take through the body.
The resistance of the human body can vary depending on factors such as skin moisture, contact area, and the path the current takes, making it difficult to accurately predict the exact current that will flow through the body based solely on the applied voltage.
Moreover, the human body is not a linear resistor; it exhibits non-linear behavior when subjected to electric currents, especially at high voltages or high frequencies.
This non-linearity is due to various physiological factors, such as the body’s capacitive and inductive properties, the presence of nerves and muscles, and the potential for tissue damage at high current levels.
In the context of electric shock, the effects on the human body are more accurately described by the concept of electrical safety thresholds and the effects of current on vital physiological functions, rather than by Ohm’s law alone.
Factors such as the current path, the duration of the shock, the frequency of the current, and the individual’s health and physical condition all play a critical role in determining the severity of the physiological response to electric shock.
Does ohm’s law change for the high or low frequencies?
Yes, Ohm’s law remains valid for both high and low frequencies in DC and AC circuits. However, at high frequencies, the behavior of circuits may be influenced by additional factors such as capacitance and inductance, which can affect the overall impedance of the circuit.
This impedance includes both the resistive component (R) and the reactive components due to capacitance (Xc) and inductance (Xl).
In AC circuits, the impedance (Z) is given by the formula:
Z=√[R2+(Xl−Xc)2]
where:
- R is the resistance,
- Xl is the inductive reactance,
- Xc is the capacitive reactance.
At high frequencies, the reactance values due to capacitance and inductance can become significant, altering the overall impedance and consequently affecting the current-voltage relationship in the circuit.
In such cases, engineers often use more complex models like the impedance triangle or phasor diagrams to analyze the behavior of the circuit accurately.
These models help in understanding the phase relationships between voltage and current in AC circuits and are essential for the design and analysis of high-frequency electronic circuits, including those used in telecommunications, radio frequency systems, and high-speed digital systems.
How can you use Ohm’s law in real-life scenarios?
Ohm’s law is widely applicable in various real-life scenarios, ranging from simple everyday situations to complex electrical engineering applications. Here are some ways Ohm’s law can be used in practical situations:
-
Household Circuits: Ohm’s law can be applied to understand the power consumption of household appliances, helping you calculate the current drawn and the power consumed by devices such as lamps, heaters, and kitchen appliances. This knowledge can assist in managing electricity usage and estimating energy costs.
-
Electronics Design and Troubleshooting: Engineers use Ohm’s law extensively in designing and troubleshooting electronic circuits. By applying Ohm’s law, they can determine the appropriate resistor values, predict the behavior of different components, and ensure that circuits function within safe operating parameters.
-
Automotive Systems: Ohm’s law is crucial in automotive systems for understanding the electrical components, such as lighting systems, batteries, and various sensors. It helps in diagnosing electrical issues and optimizing the performance of the vehicle’s electrical systems.
-
Renewable Energy Systems: In the context of renewable energy, Ohm’s law is instrumental in understanding the behavior of solar panels, wind turbines, and other energy conversion systems. It aids in designing efficient energy systems and optimizing the power output from renewable sources.
-
Industrial Applications: Ohm’s law finds applications in industrial settings for designing and maintaining electrical systems in factories and manufacturing plants. It helps engineers and technicians ensure the safe and efficient operation of machinery and equipment.
-
Safety Regulations: Understanding Ohm’s law is essential for adhering to safety regulations, especially in scenarios involving electrical safety. It helps in determining appropriate circuit protections, such as fuses and circuit breakers, to prevent electrical hazards and ensure the safety of personnel and equipment.
By applying Ohm’s law, individuals can make informed decisions, troubleshoot electrical issues, and design efficient and safe electrical systems across a wide range of applications in various industries and everyday life.
Can Ohm’s law explain what does kill “current or voltage”?
Ohm’s law by itself cannot fully explain what kills in an electric shock, as the effects of electric shock depend on various factors beyond just current or voltage, such as the duration of the shock, the path the current takes through the body, the frequency of the current, the resistance of the body, and individual physiological differences.
However, in terms of the physiological effects of an electric shock, the severity of the shock primarily depends on the current that passes through the body, rather than just the voltage. According to the concept of electrical safety, it is generally the current that poses the most significant risk to human life.
When a current passes through the human body, it can disrupt the normal functioning of the nervous system and vital organs, including the heart. Even a small current passing through the heart can lead to ventricular fibrillation, which can be fatal.
The resistance of the human body varies depending on factors such as skin condition and the path the current takes, and this influences the current flowing through the body according to Ohm’s law.
While voltage is necessary to drive current through the body, it is the current, in combination with the path it takes and the duration of exposure, that has the most significant effect on the severity of an electric shock and the potential for fatality.
Ohm’s law helps us understand the relationship between voltage, current, and resistance, but it is not sufficient on its own to fully explain the effects of electric shock on the human body.
How can the ohm’s law be used to define one ohm?
Ohm’s law can be used to define one ohm in terms of the fundamental units of voltage, current, and resistance. One ohm (1 Ω) is the amount of resistance through which one ampere of current will flow when one volt of electrical potential difference is applied across it.
Mathematically, V=IR,
R= V/I
The equation written above gives us a ratio of voltage and current. So, we have another way to state one ohm as 1 volt per unit current is 1-ohm resistance. That too is just another way to say the first sentence.
What are ohmic and non-ohmic devices?
Ohmic and non-ohmic devices refer to the behavior of materials concerning Ohm’s law, which describes the relationship between voltage, current, and resistance in a conductor.
-
Ohmic devices: Ohmic devices, or ohmic conductors, are those that follow Ohm’s law, meaning their resistance remains constant regardless of the voltage or current passing through them. The relationship between voltage and current is linear, and the resistance is independent of the voltage applied. Examples of ohmic devices include most metallic conductors such as copper wires, as long as they are within their elastic limits and operating within normal temperature ranges.
-
Non-ohmic devices: Non-ohmic devices are those that do not follow Ohm’s law and whose resistance changes with the applied voltage or current. The resistance of non-ohmic devices is not constant and may vary with factors like the temperature, the magnitude of the applied voltage, or the current passing through them. Examples of non-ohmic devices include diodes, transistors, and certain types of semiconductors. In these devices, the current-voltage relationship is not linear, and the resistance is not constant.
Understanding the distinction between ohmic and non-ohmic behavior is crucial in the field of electrical engineering and physics, as it helps in the analysis and design of various electronic circuits and devices. Differentiating between these behaviors allows for the appropriate application of Ohm’s law and the selection of suitable components for specific circuit requirements.
Install my Free Android App on Google Play:
Electrical Cables Most Common Tables “Electrical Cables Tables”
And, my Electrical Calculations App “Fast Electrical Calculator”
Discover more great content by subscribing to My channel
Looking to stay ahead of the game in the world of electrical engineering? Subscribe to my YouTube channel and gain access to exclusive content you won’t find anywhere else!
The staff I recommend
(Amazon Affiliate Links to products I believe are high quality):
- Economy 120 Volt/60Hz AC Power Source – Step-Down Voltage & Frequency Converters 1800W
- UNI-T Digital Multimeter Tester UT139C
- 50-Amp Extension Cord for RV “100ft”
- Voltage Stabilizer 110/220v
- Hair Dryer “best selling“
- TOSHIBA EM131A5C-BS Countertop Microwave Ovens
Disclaimer: This contains affiliate links to Amazon products. I may earn a commission for purchases made through these links.