An electric generator is a device that converts mechanical energy into electrical energy. It typically consists of a coil of wire (the rotor) that is rotated within a magnetic field, inducing an electric current in the wire.
This process is used to generate electricity in power plants, portable generators, and various other applications.
The output electrical energy is in the form of alternating current (AC current). Therefore, AC generators are sometimes referred to as Alternators.
The mechanical energy to the generators is provided in the form of combustion engines, steam, or gas turbines.
Table of Contents
Who invented the AC generator?
Charles F. Brush is credited as the inventor of the alternating current (AC) generator. Born on March 17, 1849, he passed away on June 15, 1929. Brush, a notable inventor and industrialist, developed an electric AC generator with adjustable voltage control based on the load and a constant current output.
Brush’s contributions extended beyond invention; he established the Brush Electric Company and served as the founding president of the Linde Air Products Company. His remarkable work in the field of electricity earned him recognition from the American Academy of Arts and Sciences, which awarded him the prestigious Rumford Medal in 1899.
In 1876, Brush secured funding from Cleveland’s Wetting Supply Company to create his “dynamo,” an early electrical generator used for arc lighting. While he initially drew inspiration from Zénobe Gramme’s dynamo design, Brush’s final creation significantly diverged, utilizing the ring armature concept pioneered by Antonio Pacinotti.
In his U.S. Patent 189,997, Brush highlighted the limitations of existing magneto-electric apparatus, which were large, heavy, expensive, and inefficient in terms of mechanical power consumption. Brush’s dynamo, after rigorous testing in 1878 by the Franklin Institute of Philadelphia, emerged as a superior alternative to the Gramme dynamo and other European counterparts. Its advantages lay in its simplified design and ease of maintenance.
Working Principle of AC Generator
An AC (alternating current) generator, often referred to as an alternator, operates on the principle of electromagnetic induction.
It is a device that converts mechanical energy into electrical energy in the form of alternating current. Here’s a simplified explanation of the working principle of an AC generator:
1. Rotational Motion: The generator is typically connected to a mechanical prime mover, such as a steam turbine, gas engine, or even a hand crank. When this prime mover is activated, it rotates a shaft.
2. Magnetic Field: Inside the generator, there are two crucial components: a stationary magnetic field and a rotating conductor (usually a coil of wire). The stationary magnetic field is typically created by permanent magnets or by using an electromagnet, which is supplied with a direct current to create a magnetic field.
3. Electromagnetic Induction: As the conductor (coil of wire) rotates within the magnetic field, the relative motion between the magnetic field and the conductor induces an electromotive force (EMF) or voltage across the ends of the coil. This phenomenon is known as electromagnetic induction, and it follows Faraday’s law of electromagnetic induction.
4. Alternating Current Output: The induced voltage across the coil’s ends causes electrons within the wire to move, creating an electric current. What makes it an alternating current generator is that as the coil continues to rotate, the direction of the induced current changes periodically. This change in direction results in an alternating current, where the electric current flows in one direction, then reverses and flows in the opposite direction, creating a sinusoidal waveform.
5. Voltage Regulation: The generator can be designed to regulate the voltage output by adjusting factors such as the strength of the magnetic field, the speed of rotation, or the number of turns in the coil. This allows for the generation of AC at a desired voltage and frequency.
An AC generator works by rotating a coil of wire within a magnetic field, which induces a
An electric generator is a device that converts mechanical energy into electrical energy. It typically consists of a coil of wire (the rotor) that is rotated within a magnetic field, inducing an electric current in the wire.
This process is used to generate electricity in power plants, portable generators, and various other applications.
The output electrical energy is in the form of alternating current (AC current). Therefore, AC generators are sometimes referred to as Alternators.
The mechanical energy to the generators is provided in the form of combustion engines, steam, or gas turbines.
Who invented the AC generator?
Charles F. Brush is credited as the inventor of the alternating current (AC) generator. Born on March 17, 1849, he passed away on June 15, 1929. Brush, a notable inventor and industrialist, developed an electric AC generator with adjustable voltage control based on the load and a constant current output.
Brush’s contributions extended beyond invention; he established the Brush Electric Company and served as the founding president of the Linde Air Products Company. His remarkable work in the field of electricity earned him recognition from the American Academy of Arts and Sciences, which awarded him the prestigious Rumford Medal in 1899.
In 1876, Brush secured funding from Cleveland’s Wetting Supply Company to create his “dynamo,” an early electrical generator used for arc lighting. While he initially drew inspiration from Zénobe Gramme’s dynamo design, Brush’s final creation significantly diverged, utilizing the ring armature concept pioneered by Antonio Pacinotti.
In his U.S. Patent 189,997, Brush highlighted the limitations of existing magneto-electric apparatus, which were large, heavy, expensive, and inefficient in terms of mechanical power consumption. Brush’s dynamo, after rigorous testing in 1878 by the Franklin Institute of Philadelphia, emerged as a superior alternative to the Gramme dynamo and other European counterparts. Its advantages lay in its simplified design and ease of maintenance.
Working Principle of AC Generator
An AC (alternating current) generator, often referred to as an alternator, operates on the principle of electromagnetic induction.
It is a device that converts mechanical energy into electrical energy in the form of alternating current. Here’s a simplified explanation of the working principle of an AC generator:
1. Rotational Motion: The generator is typically connected to a mechanical prime mover, such as a steam turbine, gas engine, or even a hand crank. When this prime mover is activated, it rotates a shaft.
2. Magnetic Field: Inside the generator, there are two crucial components: a stationary magnetic field and a rotating conductor (usually a coil of wire). The stationary magnetic field is typically created by permanent magnets or by using an electromagnet, which is supplied with a direct current to create a magnetic field.
3. Electromagnetic Induction: As the conductor (coil of wire) rotates within the magnetic field, the relative motion between the magnetic field and the conductor induces an electromotive force (EMF) or voltage across the ends of the coil. This phenomenon is known as electromagnetic induction, and it follows Faraday’s law of electromagnetic induction.
4. Alternating Current Output: The induced voltage across the coil’s ends causes electrons within the wire to move, creating an electric current. What makes it an alternating current generator is that as the coil continues to rotate, the direction of the induced current changes periodically. This change in direction results in an alternating current, where the electric current flows in one direction, then reverses and flows in the opposite direction, creating a sinusoidal waveform.
5. Voltage Regulation: The generator can be designed to regulate the voltage output by adjusting factors such as the strength of the magnetic field, the speed of rotation, or the number of turns in the coil. This allows for the generation of AC at a desired voltage and frequency.
An AC generator works by rotating a coil of wire within a magnetic field, which induces a changing voltage across the coil due to electromagnetic induction.
This changing voltage results in an alternating current output, making AC generators fundamental in generating the electricity used in homes, industries, and various electrical devices.
What are the uses of AC generators?
AC (alternating current) generators, also known as alternators, are electrical devices that convert mechanical energy into alternating current electricity. They find various applications in different industries and everyday life. Here are some common uses of AC generators:
1. Power Generation: AC generators are widely used in power plants to produce electricity for residential, commercial, and industrial purposes. They can be driven by various energy sources, such as steam, water, wind, or diesel engines, to generate electrical power.
2. Backup Power Supply: AC generators are often used as backup power sources for critical facilities like hospitals, data centers, and emergency services. They provide electricity during power outages to ensure uninterrupted operations.
3. Portable Generators: Small AC generators, often in the form of portable generators, are used for outdoor events, camping, construction sites, and other remote locations where access to the electrical grid is limited or unavailable.
4. Automotive Alternators: In automobiles, AC generators, known as alternators, play a crucial role in charging the battery and providing power to the vehicle’s electrical systems, including lights, air conditioning, and entertainment systems.
5. Aircraft Generators: Aircraft rely on AC generators to produce electrical power for onboard systems, including navigation, communication, lighting, and avionics.
6. Marine Generators: Boats and ships use AC generators to generate electricity for lighting, navigation, refrigeration, and other onboard systems.
7. Railway Electrification: AC generators are used in railway electrification systems to provide power for trains and related infrastructure. They convert mechanical energy from locomotives into electrical energy.
8. Construction Equipment: AC generators are incorporated into various construction equipment, such as welding machines, concrete mixers, and power tools, to provide electrical power at construction sites.
9. Oil and Gas Industry: AC generators are used in the oil and gas industry to power drilling rigs, pumps, and other equipment in remote locations.
10. Renewable Energy Systems: AC generators are essential components in renewable energy systems like wind turbines and hydroelectric generators. They convert the rotational energy from wind or water into electrical power.
11. Testing and Research: AC generators are used in laboratories and research facilities for experimental purposes, including testing electrical equipment, simulating various electrical conditions, and conducting experiments in electromagnetism.
12. Standalone Power Systems: In off-grid or remote areas where there is no access to the conventional electrical grid, AC generators can be used as standalone power systems, often in conjunction with energy storage solutions like batteries.
13. Entertainment and Events: AC generators are employed at outdoor concerts, festivals, and other events to provide power for stage lighting, sound systems, and other equipment.
14. Emergency Services: Emergency service vehicles, such as mobile command centers and rescue vehicles, may use AC generators to provide power for communication and equipment during emergency situations.
Overall, AC generators are versatile devices that play a critical role in generating electrical power for a wide range of applications, ensuring access to electricity in various settings and industries.
How many types of AC generators?
AC (alternating current) generators, also known as alternators, come in various types and configurations, each designed for specific applications. The main types of AC generators include:
1. Synchronous Generators: Synchronous generators are designed to produce electricity with a constant frequency, which is synchronized with the grid frequency. They are commonly used in power plants and large-scale electrical generation systems. Synchronous generators are often driven by steam turbines, gas turbines, or hydro turbines.
2. Induction Generators: Induction generators, also known as asynchronous generators, are typically used in applications where the load is variable, such as wind turbines and some small-scale power generation systems. They do not require a separate excitation source and are self-starting. Induction generators are asynchronous because their rotor speed is not precisely synchronized with the grid frequency.
3. Brushless Alternators: Brushless alternators are designed to eliminate the need for brushes and slip rings, which are common components in older generator designs. They are often used in portable and backup generators due to their reduced maintenance requirements and enhanced reliability.
4. Permanent Magnet Generators (PMGs): PMGs use permanent magnets in the rotor instead of electromagnets or field windings. They are commonly found in small-scale renewable energy systems, such as small wind turbines and portable generators. PMGs are known for their simplicity and efficiency.
5. Homopolar Generators: Homopolar generators, also called unipolar generators, are relatively rare and typically used for specialized applications like high-current, low-voltage systems. They have a simple design with a single magnetic pole and a rotating conductor.
6. Wound Rotor Generators: Wound rotor generators have a wound rotor (instead of a squirrel-cage rotor commonly found in induction motors). They are often used in specialized applications where adjustable speed and precise control of the generator’s characteristics are required.
7. Revolving Armature Generators: In revolving armature generators, the armature winding is stationary, and the magnetic field rotates around it. These generators are not commonly used in modern applications but have historical significance.
8. Inverter Generators: Inverter generators are a type of AC generator that converts the generated AC power into DC power and then inverts it back to a stable AC output. They are known for their compact size, low noise, and clean power output, making them suitable for sensitive electronic devices and portable applications.
9. Multi-Phase Generators: While most generators produce single-phase AC power, multi-phase generators can produce multiple phases of AC power, such as three-phase power commonly used in industrial applications. Multi-phase generators are essential for driving three-phase motors and equipment.
The choice of AC generator type depends on the specific requirements of the application, including the desired power output, frequency, voltage, and environmental conditions. Different generators are selected based on their efficiency, reliability, and suitability for the intended use.
Which is better synchronous or asynchronous generator?
The choice between synchronous and asynchronous (induction) generators depends on the specific application and requirements. Neither type is universally “better” than the other; instead, they have distinct advantages and disadvantages, making them more suitable for particular situations. Here’s a comparison of synchronous and asynchronous generators:
Synchronous Generators:
1. Constant Frequency: Synchronous generators produce electricity with a constant and precise frequency, which is synchronized with the grid frequency. This characteristic makes them ideal for applications where maintaining a stable and synchronized power supply is crucial, such as in large-scale power plants.
2. High Efficiency: Synchronous generators are known for their high efficiency, especially when operated at or near their synchronous speed. This makes them suitable for power generation in grid-connected systems.
3. Adjustable Power Factor: Synchronous generators allow for control of the power factor, which can be useful in stabilizing the voltage and improving power quality in electrical grids.
4. Complex Control and Excitation: Synchronous generators require complex control systems and excitation equipment to maintain synchronization with the grid and control power output. This adds to the overall system cost and complexity.
5. Suitable for Large-Scale Power Generation: Synchronous generators are commonly used in power plants, where the demand for large-scale, grid-connected electricity generation is high.
Asynchronous (Induction) Generators:
1. Self-Starting: Induction generators are self-starting, which means they do not require a separate prime mover to bring them up to speed. This makes them well-suited for applications where starting torque may be limited.
2. Robust and Low Maintenance: Induction generators have a simple design with no brushes or slip rings, leading to reduced maintenance requirements and increased reliability.
3. Variable Speed Operation: Induction generators can operate at variable speeds and do not need to maintain synchronous speed, making them suitable for applications where the load or input power varies, such as in wind turbines.
4. Less Efficient at Full Load: Induction generators tend to be less efficient than synchronous generators when operating at or near full load. This can be a disadvantage in applications where high efficiency is essential.
5. Commonly Used in Distributed Generation: Induction generators are often used in distributed generation systems, small-scale renewable energy installations, and remote areas with variable power demand.
Neither synchronous nor asynchronous generators are inherently better than the other; it depends on the specific requirements of the application. Synchronous generators excel in applications that demand precise frequency control and high efficiency, especially in large-scale power generation.
On the other hand, asynchronous generators are well-suited for applications with variable loads, and self-starting requirements, and where simplicity and robustness are essential, such as in distributed generation and some renewable energy systems. The choice should be made based on the specific needs and constraints of the project.
Why do we use synchronous generators In the Power Plant?
Synchronous generators are commonly used in power plants for several important reasons:
1. Precise Frequency Control: Synchronous generators can produce electricity with a constant and precise frequency that is synchronized with the grid frequency (e.g., 50 Hz or 60 Hz). Maintaining a stable frequency is essential for the reliable operation of electrical grids and the synchronization of various interconnected power sources.
2. Grid Compatibility: Synchronous generators are well-suited for grid-connected power generation. They can easily be synchronized with the existing grid, ensuring that the power produced is in phase with the grid’s voltage, frequency, and phase angle. This compatibility allows for efficient and reliable power transmission and distribution.
3. High Efficiency: Synchronous generators are known for their high efficiency, especially when operated near their synchronous speed. This efficiency is essential in power plants where large amounts of electricity are generated to meet the demands of residential, commercial, and industrial customers.
4. Power Factor Control: Synchronous generators offer control over the power factor, which is crucial for maintaining the voltage quality and stability of the electrical grid. By adjusting the excitation level, power plant operators can improve power factor and grid performance.
5. Large-Scale Power Generation: Synchronous generators are well-suited for large-scale power generation due to their capacity to produce significant amounts of electricity. Many power plants, such as thermal power plants, hydroelectric plants, and some nuclear power plants, require high-capacity generators to meet the electrical demands of entire regions.
6. Grid Frequency Regulation: Synchronous generators can help regulate the grid frequency by adjusting their output to match variations in electricity demand. This frequency regulation is crucial for maintaining grid stability.
7. Base Load and Peaking Power: Synchronous generators can serve as base load generators, running continuously to provide a steady supply of power. They can also be used as peaking units during periods of high demand when additional power is needed to supplement the grid.
8. Grid Voltage Support: Synchronous generators can provide reactive power support to the grid, helping to control and stabilize voltage levels. This is essential for maintaining voltage within acceptable limits.
9. Backup and Redundancy: Power plants often include multiple synchronous generators for redundancy and backup. In the event of a generator failure, others can continue to operate, ensuring a continuous power supply.
Overall, synchronous generators play a vital role in power plants due to their ability to produce stable and synchronized electricity, maintain grid stability, and provide efficient and reliable power generation for a wide range of applications.
Their characteristics make them well-suited for serving as the backbone of many electrical power systems worldwide.
For more information read my detailed article about electric generator synchronization
Is the AC generator rated in kVA or kW?
AC (alternating current) generators, whether synchronous or asynchronous, are typically rated in both kVA (kilovolt-amperes) and kW (kilowatts).
These two ratings provide essential information about the generator’s capacity and its ability to deliver real power (kW) and apparent power (kVA). Here’s a brief explanation of each rating:
1. kW (Kilowatts): This rating represents the real power output of the generator. It indicates the actual usable power that the generator can provide to electrical loads, such as lights, motors, and heating elements. Real power is what performs useful work in electrical circuits.
2. kVA (Kilovolt-Amperes): This rating represents the apparent power output of the generator. Apparent power is the combination of real power (kW) and reactive power (kVAR). Reactive power is necessary for supporting inductive loads (e.g., electric motors) and maintaining voltage levels. It does not perform useful work but is essential for the proper functioning of electrical equipment.
Generators are rated in kVA to account for both real and reactive power. The relationship between real power (kW), apparent power (kVA), and power factor (PF) is as follows:
kW = kVA × PF
Where:
– kW is the real power output in kilowatts.
– kVA is the apparent power output in kilovolt-amperes.
– PF is the power factor, which is the ratio of real power (kW) to apparent power (kVA). It is a value between 0 and 1, indicating how effectively the generator converts apparent power into real power.
In practical terms, the kW rating indicates the actual usable capacity of the generator for powering electrical loads, while the kVA rating represents the total capacity, including the ability to support reactive loads.
Both ratings are crucial for selecting a generator that can meet the power needs of specific applications and ensuring proper sizing and operation in electrical systems.
Different Parts of AC Generator
An AC (alternating current) generator, also known as an alternator, consists of various components that work together to convert mechanical energy into electrical energy. Here are the main parts of an AC generator:
1. Rotor: The rotor, also called the armature, is the rotating component of the generator. It is typically mounted on a shaft and is connected to a mechanical energy source, such as a turbine, engine, or windmill. The rotor consists of a coil or winding of wire, which rotates within the magnetic field of the stator.
2. Stator: The stator is the stationary part of the generator that surrounds the rotor. It contains the stationary coils or windings of wire and generates a magnetic field when an electric current passes through it. The interaction between the magnetic field produced by the stator and the rotating magnetic field of the rotor induces an electromotive force (EMF) in the rotor, resulting in electrical voltage generation.
3. Excitation System: The excitation system is responsible for providing the initial magnetic field in the stator. Depending on the generator type, this can be achieved using various methods, such as permanent magnets, field windings supplied with direct current (DC), or a separate exciter generator. The excitation system determines the generator’s voltage regulation and control.
4. Slip Rings and Brushes (if applicable): In some AC generators, particularly larger ones, slip rings and brushes are used to transfer electrical power from the rotor to the external load or electrical system. Slip rings are rings mounted on the rotor shaft that make electrical contact with stationary brushes, allowing the generated electricity to be collected and transmitted.
5. Terminal Box: The terminal box is the part of the generator where the electrical connections are made. It houses the output terminals through which the generated electricity is transferred to external circuits or loads.
6. Bearings and Lubrication: Bearings are used to support the rotor shaft and reduce friction during rotation. Proper lubrication is essential to ensure smooth and efficient operation of the bearings.
7. Cooling System: Many generators are equipped with a cooling system to dissipate heat generated during operation. This system can include fans, radiators, or other cooling methods, depending on the generator’s size and application.
8. Voltage Regulator and Control System: In some generators, a voltage regulator and control system are integrated to regulate the output voltage and control various parameters, such as frequency and power factor.
9. Frame and Housing: The frame provides structural support for the generator and helps protect its internal components from environmental factors. It also aids in noise reduction.
10. Protective Devices: Generators may have protective devices, such as circuit breakers, fuses, and overcurrent relays, to safeguard the generator and connected electrical systems from faults and overloads.
11. Governor (if driven by an engine): If the generator is driven by an engine, a governor may be used to control the engine’s speed and, consequently, the generator’s frequency.
These are the fundamental components of an AC generator. The specific design and configuration of these parts can vary depending on the generator’s size, type, and intended application.
Generators come in various forms, from small portable units to large industrial generators, and their components are tailored to meet the requirements of their respective applications.
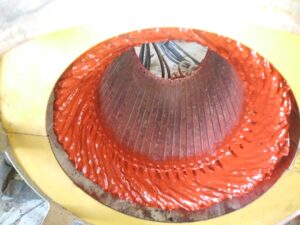
What is an AC generator exciter used for?
An AC generator exciter is used to provide the initial magnetic field necessary for the generation of electricity in an AC (alternating current) generator, also known as an alternator.
The exciter serves a critical role in the operation of the generator by establishing a magnetic field in the stator, which is the stationary part of the generator, surrounding the rotor (the rotating part). Here’s how an AC generator exciter works and its main purposes:
1. Establishing the Magnetic Field: An AC generator operates based on the principle of electromagnetic induction, where a changing magnetic field induces an electromotive force (EMF) in a coil or winding of wire. To generate electricity, the generator needs a magnetic field within the stator. The exciter is responsible for creating this initial magnetic field.
2. Field Winding: The exciter typically consists of a field winding—a coil of wire—connected to a separate power source, often a DC (direct current) power supply. When DC current flows through the field winding, it produces a magnetic field within the stator core. This magnetic field is stationary and serves as the foundation upon which the generator’s operation is based.
3. Excitation Control: The amount of DC current supplied to the field winding of the exciter can be controlled to adjust the strength of the magnetic field generated in the stator. This control allows operators to regulate the generator’s voltage output. By increasing or decreasing the excitation current, they can fine-tune the generator’s voltage as needed to match the load or grid requirements.
4. Voltage Regulation: Proper voltage regulation is essential in electrical systems to ensure that the generated voltage remains within acceptable limits, regardless of variations in load or other operating conditions. The exciter’s control system helps maintain the desired output voltage of the generator.
5. Synchronization with the Grid: In grid-connected power generation systems, the exciter is critical for synchronizing the generator’s output with the grid’s voltage and frequency. It helps ensure that the generator is operating in phase with the grid to facilitate seamless power transfer and grid stability.
6. Reactive Power Control: The excitation system can also control the generation of reactive power, which is necessary to support inductive loads and maintain voltage levels. This is particularly important in power systems with fluctuating loads and variable power factor requirements.
In summary, an AC generator exciter is a key component of the generator’s electrical system, responsible for establishing and controlling the magnetic field within the stator.
By regulating the strength of this magnetic field, the exciter influences the generator’s voltage output, synchronization with the grid, and reactive power generation.
This control is crucial for the reliable and stable operation of AC generators in various applications, including power plants, industrial facilities, and electrical grids.
AC generator protection
AC generator protection is a crucial aspect of ensuring the reliable and safe operation of alternators or generators in various applications, including power plants, industrial facilities, and emergency backup systems. Generator protection systems are designed to detect abnormal conditions, faults, and emergencies and take appropriate actions to prevent damage to the generator and the connected electrical system. Here are some common aspects of AC generator protection:
1. Overcurrent Protection: Overcurrent protection devices, such as circuit breakers and fuses, are used to safeguard the generator against excessive current flow that could lead to overheating and damage. These devices trip when the current exceeds a preset threshold.
2. Overload Protection: Overload protection prevents the generator from being overloaded for an extended period. It is designed to protect the generator from damage due to sustained high load conditions.
3. Short Circuit Protection: Short circuit protection devices, like high-speed circuit breakers, are essential for quickly disconnecting the generator from the electrical system in case of a short circuit, preventing damage to the generator and other connected equipment.
4. Earth Fault Protection: Earth fault or ground fault protection detects electrical faults to ground and isolates the generator from the system to prevent electric shock hazards and equipment damage.
5. Overvoltage and Undervoltage Protection: These protective relays monitor the generator’s voltage levels and trip the generator or activate voltage regulation systems if the voltage goes above or below predefined limits.
6. Overfrequency and Underfrequency Protection: Similar to voltage protection, these relays monitor the generator’s frequency and act if it deviates from the acceptable range, which can indicate issues like engine speed variations or loss of load.
7. Differential Protection: Differential protection compares the currents at the generator’s input and output to detect internal faults, such as winding short circuits. It is especially critical for large generators.
8. Loss of Excitation Protection: Loss of excitation protection systems detect and respond to a loss of the magnetic field (excitation) in the generator. This can happen due to various reasons and can lead to a generator trip or voltage collapse.
9. Reverse Power Protection: Reverse power protection prevents the generator from operating as a motor when power flows in the reverse direction, which can occur during certain fault conditions.
10. Overtemperature Protection: Generators can overheat due to sustained high loads or inadequate cooling. Temperature sensors and protective devices are used to monitor and control generator temperatures.
11. Synchronization Protection: In grid-connected systems, synchronization protection ensures that the generator synchronizes properly with the grid before being connected, preventing phase mismatches and damage.
12. Emergency Shutdown: In some cases, emergency shutdown systems can be activated in response to critical issues, such as catastrophic failures, fire, or safety concerns.
13. Generator Grounding: Proper grounding and grounding protection are essential for safety and to prevent electrical faults.
Generator protection systems typically include a combination of relays, sensors, alarms, and automated controls to detect and respond to abnormal conditions promptly.
These systems are designed to minimize downtime, protect equipment, and ensure the safe and reliable operation of the generator and the connected electrical system.
The specific protection scheme and devices used depend on the generator’s type, size, and application.
Can I Use a Stabilizer with a Generator?
Yes, you can use a voltage stabilizer or voltage regulator with a generator to help maintain a stable and consistent voltage output.
Generators, especially smaller portable units, may experience fluctuations in voltage and frequency due to varying loads, engine speed changes, or other factors.
Voltage stabilizers or regulators can help mitigate these fluctuations and provide a more reliable and consistent power supply. Here’s how they work and why you might consider using them:
Voltage Stabilizer/Regulator: A voltage stabilizer (also known as an automatic voltage regulator or AVR) is an electrical device designed to control the output voltage of a generator or other power source.
It does this by automatically adjusting the excitation current supplied to the generator’s field winding. By doing so, it keeps the output voltage within a specified range, typically very close to the desired nominal voltage (e.g., 230V or 120V).
Benefits of Using a Voltage Stabilizer with a Generator:
a. Voltage Regulation: The primary benefit is voltage regulation, ensuring that the output voltage remains stable, regardless of changes in the generator’s load or operating conditions. This is important for protecting sensitive electrical equipment from voltage fluctuations.
b. Equipment Protection: Voltage stabilizers help prevent overvoltage and under-voltage conditions, which can damage or degrade electrical appliances and machinery. They also provide a consistent voltage supply, extending the lifespan of connected equipment.
c. Improved Power Quality: Stabilizers improve the overall quality of the power supply by reducing voltage sags, surges, and fluctuations, which can cause operational issues and downtime in industrial processes.
d. Compatibility: Voltage stabilizers are compatible with a wide range of generator types and sizes, from small portable units to larger industrial generators.
Using a voltage stabilizer or voltage regulator with a generator is a practical way to enhance the quality and reliability of the generated power.
It helps protect sensitive equipment, maintains voltage within acceptable limits, and ensures consistent and stable electrical output, which is crucial in various applications and industries.
advantages and disadvantages of AC generators
AC (alternating current) generators, also known as alternators, have both advantages and disadvantages, depending on the application and specific requirements. Here’s a summary of the key advantages and disadvantages of AC generators:
Advantages of AC Generators:
1. Compatibility with the Grid: AC generators are inherently compatible with the electrical grid, making them suitable for grid-connected power generation and synchronization.
2. Constant Frequency: AC generators can produce electricity with a constant and precise frequency, making them ideal for applications that require stable frequency output, such as power plants.
3. Efficient Transmission: AC power is well-suited for long-distance transmission, thanks to the ease of voltage transformation using transformers, which reduces losses during transmission.
4. Voltage Regulation: AC generators can be equipped with voltage regulators and control systems to maintain a stable voltage output, ensuring reliable power quality.
5. Induction Motors: AC generators can directly power induction motors, which are widely used in industrial applications.
6. Ease of Synchronization: AC generators can be easily synchronized with other generators or the grid, allowing for parallel operation and load sharing.
7. No Commutation: Unlike DC generators, AC generators do not require commutators or brushes, resulting in reduced maintenance and longer operational life.
8. Wide Range of Sizes: AC generators are available in a wide range of sizes and capacities, from small portable units to large industrial generators.
9. Variable Speed Operation: AC generators can operate at variable speeds without affecting frequency, making them suitable for variable-speed prime movers like wind turbines.
Disadvantages of AC Generators:
1. Complex Control Systems: Synchronous AC generators require complex control systems to maintain synchronization with the grid and control power output.
2. Initial Excitation: AC generators require an initial source of excitation (magnetic field) to start generating electricity. This can be provided by permanent magnets, field windings, or separate exciter generators.
3. Synchronous Speed: Synchronous AC generators must operate at a constant synchronous speed to maintain grid synchronization, limiting their flexibility in variable-speed applications.
4. Reactive Power: AC generators produce both real power (kW) and reactive power (kVAR). Managing and controlling reactive power can be challenging in some applications.
5. Mechanical Wear: Although AC generators have fewer moving parts than some other types of generators, they still experience mechanical wear over time, requiring maintenance and occasional overhauls.
6. Inefficiency at Partial Load: AC generators may be less efficient when operated at partial load, which can result in wasted energy in certain situations.
7. Sensitive to Voltage and Frequency Variations: Some sensitive electronic equipment may require additional conditioning to handle minor voltage and frequency variations that can occur in AC power systems.
The suitability of AC generators depends on the specific requirements of the application. They are widely used in power generation, industrial processes, and a variety of other settings due to their compatibility with the grid and stable frequency output.
However, they may not be the best choice for all situations, particularly those with variable-speed requirements or where DC power is preferred.
Why does an AC generator need force to run?
An AC (alternating current) generator requires an external force, typically provided by a mechanical prime mover, to run because it operates on the principle of electromagnetic induction.
In electromagnetic induction, a changing magnetic field induces an electromotive force (EMF) or voltage in a conductor (such as a coil of wire). To generate electricity in an AC generator, you need the following key elements:
1. Magnetic Field: An existing magnetic field is required to induce voltage in the conductor. In an AC generator, this magnetic field is usually created by one of the following methods:
– Permanent Magnets: Some small generators use permanent magnets as the source of the magnetic field. These magnets provide a fixed magnetic field without requiring external power.
– Electromagnets or Field Windings: Larger generators use either electromagnets or field windings. These are coils of wire supplied with direct current (DC) from an external source. When energized, they create a magnetic field within the generator.
2. Conductor (Rotor): A conductor, typically in the form of a coil of wire, is mounted on a rotor that can rotate within the magnetic field. As the rotor turns, the conductor experiences a changing magnetic field, which induces an EMF or voltage in the coil.
3. External Force: To make the rotor and conductor rotate within the magnetic field, you need an external force. This force is provided by a mechanical prime mover, which can be a steam turbine, gas turbine, water turbine, diesel engine, wind turbine, or any other source of mechanical energy. The prime mover’s mechanical energy is converted into rotational motion, driving the rotor and conductor.
4. Load: Once voltage is generated in the conductor, it can be connected to an electrical load, such as lights, motors, or appliances. The load consumes the electrical energy produced by the generator.
An AC generator requires an external force to run because it needs to create a changing magnetic field by rotating a conductor within an existing magnetic field.
This rotation, induced by a mechanical prime mover, is what allows the generator to generate alternating current (AC) electricity.
Without the external force, there would be no relative motion between the conductor and the magnetic field, and no voltage would be induced in the conductor, resulting in no electrical output from the generator.
Why are AC generators used over DC generators?
AC (alternating current) generators are often preferred over DC (direct current) generators in many applications due to several key advantages, including:
1. Ease of Voltage Transformation: AC voltage can be easily transformed using transformers, which allows for efficient long-distance transmission of electricity and voltage conversion to meet various load requirements. DC voltage transformation is less efficient and typically requires more complex conversion equipment.
2. Compatibility with the Grid: AC generators can be easily synchronized with an existing AC electrical grid, making them well-suited for grid-connected power generation and distribution. DC generators require additional equipment and synchronization methods to integrate with AC grids.
3. Constant Frequency: AC generators can produce electricity with a constant and precise frequency, which is essential for maintaining the synchronization of interconnected power sources and electrical equipment. Many industrial and commercial processes rely on stable AC frequency.
4. High Voltage Generation: AC generators are well-suited for high-voltage generation, which is often required for long-distance transmission and efficient power distribution.
5. Simple Commutation: AC generators do not require commutators or brushes for voltage conversion, reducing maintenance requirements and operational complexity compared to DC generators.
6. Variable Speed Operation: AC generators can operate at variable speeds without affecting the frequency of the generated power. This flexibility is valuable in applications where variable-speed prime movers are used, such as wind turbines.
7. Compatibility with Induction Motors: AC generators can directly power induction motors, which are widely used in industrial applications. This simplifies power distribution in many industrial settings.
8. No Electrolysis Issues: DC generators can produce electrolysis when used in certain applications, such as electrolysis cells or underwater applications, which can lead to equipment corrosion and other issues. AC generators do not have this problem.
9. Historical Adoption: AC power generation and distribution systems gained widespread adoption during the late 19th century, partly due to the pioneering work of inventors like Nikola Tesla and the support of figures like George Westinghouse. This historical momentum contributed to the dominance of AC systems in many parts of the world.
While AC generators offer numerous advantages, it’s important to note that DC generators still have specific applications where they excel. For example, DC generators are commonly used in applications like battery charging, electroplating, and certain industrial processes that require precise control of voltage and current.
Ultimately, the choice between AC and DC generators depends on the specific requirements of the application, including voltage needs, load types, and the availability of an existing electrical grid. Modern technology has also made it possible to convert between AC and DC power efficiently, allowing for the integration of both types of generators into diverse electrical systems
changing voltage across the coil due to electromagnetic induction.
This changing voltage results in an alternating current output, making AC generators fundamental in generating the electricity used in homes, industries, and various electrical devices.
What are the uses of AC generators?
AC (alternating current) generators, also known as alternators, are electrical devices that convert mechanical energy into alternating current electricity. They find various applications in different industries and everyday life. Here are some common uses of AC generators:
1. Power Generation: AC generators are widely used in power plants to produce electricity for residential, commercial, and industrial purposes. They can be driven by various energy sources, such as steam, water, wind, or diesel engines, to generate electrical power.
2. Backup Power Supply: AC generators are often used as backup power sources for critical facilities like hospitals, data centers, and emergency services. They provide electricity during power outages to ensure uninterrupted operations.
3. Portable Generators: Small AC generators, often in the form of portable generators, are used for outdoor events, camping, construction sites, and other remote locations where access to the electrical grid is limited or unavailable.
4. Automotive Alternators: In automobiles, AC generators, known as alternators, play a crucial role in charging the battery and providing power to the vehicle’s electrical systems, including lights, air conditioning, and entertainment systems.
5. Aircraft Generators: Aircraft rely on AC generators to produce electrical power for onboard systems, including navigation, communication, lighting, and avionics.
6. Marine Generators: Boats and ships use AC generators to generate electricity for lighting, navigation, refrigeration, and other onboard systems.
7. Railway Electrification: AC generators are used in railway electrification systems to provide power for trains and related infrastructure. They convert mechanical energy from locomotives into electrical energy.
8. Construction Equipment: AC generators are incorporated into various construction equipment, such as welding machines, concrete mixers, and power tools, to provide electrical power at construction sites.
9. Oil and Gas Industry: AC generators are used in the oil and gas industry to power drilling rigs, pumps, and other equipment in remote locations.
10. Renewable Energy Systems: AC generators are essential components in renewable energy systems like wind turbines and hydroelectric generators. They convert the rotational energy from wind or water into electrical power.
11. Testing and Research: AC generators are used in laboratories and research facilities for experimental purposes, including testing electrical equipment, simulating various electrical conditions, and conducting experiments in electromagnetism.
12. Standalone Power Systems: In off-grid or remote areas where there is no access to the conventional electrical grid, AC generators can be used as standalone power systems, often in conjunction with energy storage solutions like batteries.
13. Entertainment and Events: AC generators are employed at outdoor concerts, festivals, and other events to provide power for stage lighting, sound systems, and other equipment.
14. Emergency Services: Emergency service vehicles, such as mobile command centers and rescue vehicles, may use AC generators to provide power for communication and equipment during emergency situations.
Overall, AC generators are versatile devices that play a critical role in generating electrical power for a wide range of applications, ensuring access to electricity in various settings and industries.
How many types of AC generators?
AC (alternating current) generators, also known as alternators, come in various types and configurations, each designed for specific applications. The main types of AC generators include:
1. Synchronous Generators: Synchronous generators are designed to produce electricity with a constant frequency, which is synchronized with the grid frequency. They are commonly used in power plants and large-scale electrical generation systems. Synchronous generators are often driven by steam turbines, gas turbines, or hydro turbines.
2. Induction Generators: Induction generators, also known as asynchronous generators, are typically used in applications where the load is variable, such as wind turbines and some small-scale power generation systems. They do not require a separate excitation source and are self-starting. Induction generators are asynchronous because their rotor speed is not precisely synchronized with the grid frequency.
3. Brushless Alternators: Brushless alternators are designed to eliminate the need for brushes and slip rings, which are common components in older generator designs. They are often used in portable and backup generators due to their reduced maintenance requirements and enhanced reliability.
4. Permanent Magnet Generators (PMGs): PMGs use permanent magnets in the rotor instead of electromagnets or field windings. They are commonly found in small-scale renewable energy systems, such as small wind turbines and portable generators. PMGs are known for their simplicity and efficiency.
5. Homopolar Generators: Homopolar generators, also called unipolar generators, are relatively rare and typically used for specialized applications like high-current, low-voltage systems. They have a simple design with a single magnetic pole and a rotating conductor.
6. Wound Rotor Generators: Wound rotor generators have a wound rotor (instead of a squirrel-cage rotor commonly found in induction motors). They are often used in specialized applications where adjustable speed and precise control of the generator’s characteristics are required.
7. Revolving Armature Generators: In revolving armature generators, the armature winding is stationary, and the magnetic field rotates around it. These generators are not commonly used in modern applications but have historical significance.
8. Inverter Generators: Inverter generators are a type of AC generator that converts the generated AC power into DC power and then inverts it back to a stable AC output. They are known for their compact size, low noise, and clean power output, making them suitable for sensitive electronic devices and portable applications.
9. Multi-Phase Generators: While most generators produce single-phase AC power, multi-phase generators can produce multiple phases of AC power, such as three-phase power commonly used in industrial applications. Multi-phase generators are essential for driving three-phase motors and equipment.
The choice of AC generator type depends on the specific requirements of the application, including the desired power output, frequency, voltage, and environmental conditions. Different generators are selected based on their efficiency, reliability, and suitability for the intended use.
Which is better synchronous or asynchronous generator?
The choice between synchronous and asynchronous (induction) generators depends on the specific application and requirements. Neither type is universally “better” than the other; instead, they have distinct advantages and disadvantages, making them more suitable for particular situations. Here’s a comparison of synchronous and asynchronous generators:
Synchronous Generators:
1. Constant Frequency: Synchronous generators produce electricity with a constant and precise frequency, which is synchronized with the grid frequency. This characteristic makes them ideal for applications where maintaining a stable and synchronized power supply is crucial, such as in large-scale power plants.
2. High Efficiency: Synchronous generators are known for their high efficiency, especially when operated at or near their synchronous speed. This makes them suitable for power generation in grid-connected systems.
3. Adjustable Power Factor: Synchronous generators allow for control of the power factor, which can be useful in stabilizing the voltage and improving power quality in electrical grids.
4. Complex Control and Excitation: Synchronous generators require complex control systems and excitation equipment to maintain synchronization with the grid and control power output. This adds to the overall system cost and complexity.
5. Suitable for Large-Scale Power Generation: Synchronous generators are commonly used in power plants, where the demand for large-scale, grid-connected electricity generation is high.
Asynchronous (Induction) Generators:
1. Self-Starting: Induction generators are self-starting, which means they do not require a separate prime mover to bring them up to speed. This makes them well-suited for applications where starting torque may be limited.
2. Robust and Low Maintenance: Induction generators have a simple design with no brushes or slip rings, leading to reduced maintenance requirements and increased reliability.
3. Variable Speed Operation: Induction generators can operate at variable speeds and do not need to maintain synchronous speed, making them suitable for applications where the load or input power varies, such as in wind turbines.
4. Less Efficient at Full Load: Induction generators tend to be less efficient than synchronous generators when operating at or near full load. This can be a disadvantage in applications where high efficiency is essential.
5. Commonly Used in Distributed Generation: Induction generators are often used in distributed generation systems, small-scale renewable energy installations, and remote areas with variable power demand.
Neither synchronous nor asynchronous generators are inherently better than the other; it depends on the specific requirements of the application. Synchronous generators excel in applications that demand precise frequency control and high efficiency, especially in large-scale power generation.
On the other hand, asynchronous generators are well-suited for applications with variable loads, and self-starting requirements, and where simplicity and robustness are essential, such as in distributed generation and some renewable energy systems. The choice should be made based on the specific needs and constraints of the project.
Why do we use synchronous generators In the Power Plant?
Synchronous generators are commonly used in power plants for several important reasons:
1. Precise Frequency Control: Synchronous generators can produce electricity with a constant and precise frequency that is synchronized with the grid frequency (e.g., 50 Hz or 60 Hz). Maintaining a stable frequency is essential for the reliable operation of electrical grids and the synchronization of various interconnected power sources.
2. Grid Compatibility: Synchronous generators are well-suited for grid-connected power generation. They can easily be synchronized with the existing grid, ensuring that the power produced is in phase with the grid’s voltage, frequency, and phase angle. This compatibility allows for efficient and reliable power transmission and distribution.
3. High Efficiency: Synchronous generators are known for their high efficiency, especially when operated near their synchronous speed. This efficiency is essential in power plants where large amounts of electricity are generated to meet the demands of residential, commercial, and industrial customers.
4. Power Factor Control: Synchronous generators offer control over the power factor, which is crucial for maintaining the voltage quality and stability of the electrical grid. By adjusting the excitation level, power plant operators can improve power factor and grid performance.
5. Large-Scale Power Generation: Synchronous generators are well-suited for large-scale power generation due to their capacity to produce significant amounts of electricity. Many power plants, such as thermal power plants, hydroelectric plants, and some nuclear power plants, require high-capacity generators to meet the electrical demands of entire regions.
6. Grid Frequency Regulation: Synchronous generators can help regulate the grid frequency by adjusting their output to match variations in electricity demand. This frequency regulation is crucial for maintaining grid stability.
7. Base Load and Peaking Power: Synchronous generators can serve as base load generators, running continuously to provide a steady supply of power. They can also be used as peaking units during periods of high demand when additional power is needed to supplement the grid.
8. Grid Voltage Support: Synchronous generators can provide reactive power support to the grid, helping to control and stabilize voltage levels. This is essential for maintaining voltage within acceptable limits.
9. Backup and Redundancy: Power plants often include multiple synchronous generators for redundancy and backup. In the event of a generator failure, others can continue to operate, ensuring a continuous power supply.
Overall, synchronous generators play a vital role in power plants due to their ability to produce stable and synchronized electricity, maintain grid stability, and provide efficient and reliable power generation for a wide range of applications.
Their characteristics make them well-suited for serving as the backbone of many electrical power systems worldwide.
For more information read my detailed article about electric generator synchronization
Is the AC generator rated in kVA or kW?
AC (alternating current) generators, whether synchronous or asynchronous, are typically rated in both kVA (kilovolt-amperes) and kW (kilowatts).
These two ratings provide essential information about the generator’s capacity and its ability to deliver real power (kW) and apparent power (kVA). Here’s a brief explanation of each rating:
1. kW (Kilowatts): This rating represents the real power output of the generator. It indicates the actual usable power that the generator can provide to electrical loads, such as lights, motors, and heating elements. Real power is what performs useful work in electrical circuits.
2. kVA (Kilovolt-Amperes): This rating represents the apparent power output of the generator. Apparent power is the combination of real power (kW) and reactive power (kVAR). Reactive power is necessary for supporting inductive loads (e.g., electric motors) and maintaining voltage levels. It does not perform useful work but is essential for the proper functioning of electrical equipment.
Generators are rated in kVA to account for both real and reactive power. The relationship between real power (kW), apparent power (kVA), and power factor (PF) is as follows:
kW = kVA × PF
Where:
– kW is the real power output in kilowatts.
– kVA is the apparent power output in kilovolt-amperes.
– PF is the power factor, which is the ratio of real power (kW) to apparent power (kVA). It is a value between 0 and 1, indicating how effectively the generator converts apparent power into real power.
In practical terms, the kW rating indicates the actual usable capacity of the generator for powering electrical loads, while the kVA rating represents the total capacity, including the ability to support reactive loads.
Both ratings are crucial for selecting a generator that can meet the power needs of specific applications and ensuring proper sizing and operation in electrical systems.
Different Parts of AC Generator
An AC (alternating current) generator, also known as an alternator, consists of various components that work together to convert mechanical energy into electrical energy. Here are the main parts of an AC generator:
1. Rotor: The rotor, also called the armature, is the rotating component of the generator. It is typically mounted on a shaft and is connected to a mechanical energy source, such as a turbine, engine, or windmill. The rotor consists of a coil or winding of wire, which rotates within the magnetic field of the stator.
2. Stator: The stator is the stationary part of the generator that surrounds the rotor. It contains the stationary coils or windings of wire and generates a magnetic field when an electric current passes through it. The interaction between the magnetic field produced by the stator and the rotating magnetic field of the rotor induces an electromotive force (EMF) in the rotor, resulting in electrical voltage generation.
3. Excitation System: The excitation system is responsible for providing the initial magnetic field in the stator. Depending on the generator type, this can be achieved using various methods, such as permanent magnets, field windings supplied with direct current (DC), or a separate exciter generator. The excitation system determines the generator’s voltage regulation and control.
4. Slip Rings and Brushes (if applicable): In some AC generators, particularly larger ones, slip rings and brushes are used to transfer electrical power from the rotor to the external load or electrical system. Slip rings are rings mounted on the rotor shaft that make electrical contact with stationary brushes, allowing the generated electricity to be collected and transmitted.
5. Terminal Box: The terminal box is the part of the generator where the electrical connections are made. It houses the output terminals through which the generated electricity is transferred to external circuits or loads.
6. Bearings and Lubrication: Bearings are used to support the rotor shaft and reduce friction during rotation. Proper lubrication is essential to ensure smooth and efficient operation of the bearings.
7. Cooling System: Many generators are equipped with a cooling system to dissipate heat generated during operation. This system can include fans, radiators, or other cooling methods, depending on the generator’s size and application.
8. Voltage Regulator and Control System: In some generators, a voltage regulator and control system are integrated to regulate the output voltage and control various parameters, such as frequency and power factor.
9. Frame and Housing: The frame provides structural support for the generator and helps protect its internal components from environmental factors. It also aids in noise reduction.
10. Protective Devices: Generators may have protective devices, such as circuit breakers, fuses, and overcurrent relays, to safeguard the generator and connected electrical systems from faults and overloads.
11. Governor (if driven by an engine): If the generator is driven by an engine, a governor may be used to control the engine’s speed and, consequently, the generator’s frequency.
These are the fundamental components of an AC generator. The specific design and configuration of these parts can vary depending on the generator’s size, type, and intended application.
Generators come in various forms, from small portable units to large industrial generators, and their components are tailored to meet the requirements of their respective applications.
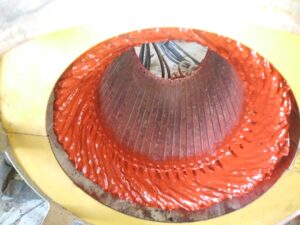
What is an AC generator exciter used for?
An AC generator exciter is used to provide the initial magnetic field necessary for the generation of electricity in an AC (alternating current) generator, also known as an alternator.
The exciter serves a critical role in the operation of the generator by establishing a magnetic field in the stator, which is the stationary part of the generator, surrounding the rotor (the rotating part). Here’s how an AC generator exciter works and its main purposes:
1. Establishing the Magnetic Field: An AC generator operates based on the principle of electromagnetic induction, where a changing magnetic field induces an electromotive force (EMF) in a coil or winding of wire. To generate electricity, the generator needs a magnetic field within the stator. The exciter is responsible for creating this initial magnetic field.
2. Field Winding: The exciter typically consists of a field winding—a coil of wire—connected to a separate power source, often a DC (direct current) power supply. When DC current flows through the field winding, it produces a magnetic field within the stator core. This magnetic field is stationary and serves as the foundation upon which the generator’s operation is based.
3. Excitation Control: The amount of DC current supplied to the field winding of the exciter can be controlled to adjust the strength of the magnetic field generated in the stator. This control allows operators to regulate the generator’s voltage output. By increasing or decreasing the excitation current, they can fine-tune the generator’s voltage as needed to match the load or grid requirements.
4. Voltage Regulation: Proper voltage regulation is essential in electrical systems to ensure that the generated voltage remains within acceptable limits, regardless of variations in load or other operating conditions. The exciter’s control system helps maintain the desired output voltage of the generator.
5. Synchronization with the Grid: In grid-connected power generation systems, the exciter is critical for synchronizing the generator’s output with the grid’s voltage and frequency. It helps ensure that the generator is operating in phase with the grid to facilitate seamless power transfer and grid stability.
6. Reactive Power Control: The excitation system can also control the generation of reactive power, which is necessary to support inductive loads and maintain voltage levels. This is particularly important in power systems with fluctuating loads and variable power factor requirements.
In summary, an AC generator exciter is a key component of the generator’s electrical system, responsible for establishing and controlling the magnetic field within the stator.
By regulating the strength of this magnetic field, the exciter influences the generator’s voltage output, synchronization with the grid, and reactive power generation.
This control is crucial for the reliable and stable operation of AC generators in various applications, including power plants, industrial facilities, and electrical grids.
AC generator protection
AC generator protection is a crucial aspect of ensuring the reliable and safe operation of alternators or generators in various applications, including power plants, industrial facilities, and emergency backup systems.
Generator protection systems are designed to detect abnormal conditions, faults, and emergencies and take appropriate actions to prevent damage to the generator and the connected electrical system. Here are some common aspects of AC generator protection:
1. Overcurrent Protection: Overcurrent protection devices, such as circuit breakers and fuses, are used to safeguard the generator against excessive current flow that could lead to overheating and damage. These devices trip when the current exceeds a preset threshold.
2. Overload Protection: Overload protection prevents the generator from being overloaded for an extended period. It is designed to protect the generator from damage due to sustained high load conditions.
3. Short Circuit Protection: Short circuit protection devices, like high-speed circuit breakers, are essential for quickly disconnecting the generator from the electrical system in case of a short circuit, preventing damage to the generator and other connected equipment.
4. Earth Fault Protection: Earth fault or ground fault protection detects electrical faults to ground and isolates the generator from the system to prevent electric shock hazards and equipment damage.
5. Overvoltage and Undervoltage Protection: These protective relays monitor the generator’s voltage levels and trip the generator or activate voltage regulation systems if the voltage goes above or below predefined limits.
6. Overfrequency and Underfrequency Protection: Similar to voltage protection, these relays monitor the generator’s frequency and act if it deviates from the acceptable range, which can indicate issues like engine speed variations or loss of load.
7. Differential Protection: Differential protection compares the currents at the generator’s input and output to detect internal faults, such as winding short circuits. It is especially critical for large generators.
8. Loss of Excitation Protection: Loss of excitation protection systems detect and respond to a loss of the magnetic field (excitation) in the generator. This can happen due to various reasons and can lead to a generator trip or voltage collapse.
9. Reverse Power Protection: Reverse power protection prevents the generator from operating as a motor when power flows in the reverse direction, which can occur during certain fault conditions.
10. Overtemperature Protection: Generators can overheat due to sustained high loads or inadequate cooling. Temperature sensors and protective devices are used to monitor and control generator temperatures.
11. Synchronization Protection: In grid-connected systems, synchronization protection ensures that the generator synchronizes properly with the grid before being connected, preventing phase mismatches and damage.
12. Emergency Shutdown: In some cases, emergency shutdown systems can be activated in response to critical issues, such as catastrophic failures, fire, or safety concerns.
13. Generator Grounding: Proper grounding and grounding protection are essential for safety and to prevent electrical faults.
Generator protection systems typically include a combination of relays, sensors, alarms, and automated controls to detect and respond to abnormal conditions promptly.
These systems are designed to minimize downtime, protect equipment, and ensure the safe and reliable operation of the generator and the connected electrical system.
The specific protection scheme and devices used depend on the generator’s type, size, and application.
Can I Use a Stabilizer with a Generator?
Yes, you can use a voltage stabilizer or voltage regulator with a generator to help maintain a stable and consistent voltage output.
Generators, especially smaller portable units, may experience fluctuations in voltage and frequency due to varying loads, engine speed changes, or other factors.
Voltage stabilizers or regulators can help mitigate these fluctuations and provide a more reliable and consistent power supply. Here’s how they work and why you might consider using them:
Voltage Stabilizer/Regulator: A voltage stabilizer (also known as an automatic voltage regulator or AVR) is an electrical device designed to control the output voltage of a generator or other power source.
It does this by automatically adjusting the excitation current supplied to the generator’s field winding. By doing so, it keeps the output voltage within a specified range, typically very close to the desired nominal voltage (e.g., 230V or 120V).
Benefits of Using a Voltage Stabilizer with a Generator:
a. Voltage Regulation: The primary benefit is voltage regulation, ensuring that the output voltage remains stable, regardless of changes in the generator’s load or operating conditions. This is important for protecting sensitive electrical equipment from voltage fluctuations.
b. Equipment Protection: Voltage stabilizers help prevent overvoltage and undervoltage conditions, which can damage or degrade electrical appliances and machinery. They also provide a consistent voltage supply, extending the lifespan of connected equipment.
c. Improved Power Quality: Stabilizers improve the overall quality of the power supply by reducing voltage sags, surges, and fluctuations, which can cause operational issues and downtime in industrial processes.
d. Compatibility: Voltage stabilizers are compatible with a wide range of generator types and sizes, from small portable units to larger industrial generators.
Using a voltage stabilizer or voltage regulator with a generator is a practical way to enhance the quality and reliability of the generated power.
It helps protect sensitive equipment, maintains voltage within acceptable limits, and ensures consistent and stable electrical output, which is crucial in various applications and industries.
advantages and disadvantages of AC generators
AC (alternating current) generators, also known as alternators, have both advantages and disadvantages, depending on the application and specific requirements. Here’s a summary of the key advantages and disadvantages of AC generators:
Advantages of AC Generators:
1. Compatibility with the Grid: AC generators are inherently compatible with the electrical grid, making them suitable for grid-connected power generation and synchronization.
2. Constant Frequency: AC generators can produce electricity with a constant and precise frequency, making them ideal for applications that require stable frequency output, such as power plants.
3. Efficient Transmission: AC power is well-suited for long-distance transmission, thanks to the ease of voltage transformation using transformers, which reduces losses during transmission.
4. Voltage Regulation: AC generators can be equipped with voltage regulators and control systems to maintain a stable voltage output, ensuring reliable power quality.
5. Induction Motors: AC generators can directly power induction motors, which are widely used in industrial applications.
6. Ease of Synchronization: AC generators can be easily synchronized with other generators or the grid, allowing for parallel operation and load sharing.
7. No Commutation: Unlike DC generators, AC generators do not require commutators or brushes, resulting in reduced maintenance and longer operational life.
8. Wide Range of Sizes: AC generators are available in a wide range of sizes and capacities, from small portable units to large industrial generators.
9. Variable Speed Operation: AC generators can operate at variable speeds without affecting frequency, making them suitable for variable-speed prime movers like wind turbines.
Disadvantages of AC Generators:
1. Complex Control Systems: Synchronous AC generators require complex control systems to maintain synchronization with the grid and control power output.
2. Initial Excitation: AC generators require an initial source of excitation (magnetic field) to start generating electricity. This can be provided by permanent magnets, field windings, or separate exciter generators.
3. Synchronous Speed: Synchronous AC generators must operate at a constant synchronous speed to maintain grid synchronization, limiting their flexibility in variable-speed applications.
4. Reactive Power: AC generators produce both real power (kW) and reactive power (kVAR). Managing and controlling reactive power can be challenging in some applications.
5. Mechanical Wear: Although AC generators have fewer moving parts than some other types of generators, they still experience mechanical wear over time, requiring maintenance and occasional overhauls.
6. Inefficiency at Partial Load: AC generators may be less efficient when operated at partial load, which can result in wasted energy in certain situations.
7. Sensitive to Voltage and Frequency Variations: Some sensitive electronic equipment may require additional conditioning to handle minor voltage and frequency variations that can occur in AC power systems.
The suitability of AC generators depends on the specific requirements of the application. They are widely used in power generation, industrial processes, and a variety of other settings due to their compatibility with the grid and stable frequency output.
However, they may not be the best choice for all situations, particularly those with variable-speed requirements or where DC power is preferred.
Why does an AC generator need force to run?
An AC (alternating current) generator requires an external force, typically provided by a mechanical prime mover, to run because it operates on the principle of electromagnetic induction.
In electromagnetic induction, a changing magnetic field induces an electromotive force (EMF) or voltage in a conductor (such as a coil of wire). To generate electricity in an AC generator, you need the following key elements:
1. Magnetic Field: An existing magnetic field is required to induce voltage in the conductor. In an AC generator, this magnetic field is usually created by one of the following methods:
– Permanent Magnets: Some small generators use permanent magnets as the source of the magnetic field. These magnets provide a fixed magnetic field without requiring external power.
– Electromagnets or Field Windings: Larger generators use either electromagnets or field windings. These are coils of wire supplied with direct current (DC) from an external source. When energized, they create a magnetic field within the generator.
2. Conductor (Rotor): A conductor, typically in the form of a coil of wire, is mounted on a rotor that can rotate within the magnetic field. As the rotor turns, the conductor experiences a changing magnetic field, which induces an EMF or voltage in the coil.
3. External Force: To make the rotor and conductor rotate within the magnetic field, you need an external force. This force is provided by a mechanical prime mover, which can be a steam turbine, gas turbine, water turbine, diesel engine, wind turbine, or any other source of mechanical energy. The prime mover’s mechanical energy is converted into rotational motion, driving the rotor and conductor.
4. Load: Once voltage is generated in the conductor, it can be connected to an electrical load, such as lights, motors, or appliances. The load consumes the electrical energy produced by the generator.
An AC generator requires an external force to run because it needs to create a changing magnetic field by rotating a conductor within an existing magnetic field.
This rotation, induced by a mechanical prime mover, is what allows the generator to generate alternating current (AC) electricity.
Without the external force, there would be no relative motion between the conductor and the magnetic field, and no voltage would be induced in the conductor, resulting in no electrical output from the generator.
Why are AC generators used over DC generators?
AC (alternating current) generators are often preferred over DC (direct current) generators in many applications due to several key advantages, including:
1. Ease of Voltage Transformation: AC voltage can be easily transformed using transformers, which allows for efficient long-distance transmission of electricity and voltage conversion to meet various load requirements. DC voltage transformation is less efficient and typically requires more complex conversion equipment.
2. Compatibility with the Grid: AC generators can be easily synchronized with an existing AC electrical grid, making them well-suited for grid-connected power generation and distribution. DC generators require additional equipment and synchronization methods to integrate with AC grids.
3. Constant Frequency: AC generators can produce electricity with a constant and precise frequency, which is essential for maintaining the synchronization of interconnected power sources and electrical equipment. Many industrial and commercial processes rely on stable AC frequency.
4. High Voltage Generation: AC generators are well-suited for high-voltage generation, which is often required for long-distance transmission and efficient power distribution.
5. Simple Commutation: AC generators do not require commutators or brushes for voltage conversion, reducing maintenance requirements and operational complexity compared to DC generators.
6. Variable Speed Operation: AC generators can operate at variable speeds without affecting the frequency of the generated power. This flexibility is valuable in applications where variable-speed prime movers are used, such as wind turbines.
7. Compatibility with Induction Motors: AC generators can directly power induction motors, which are widely used in industrial applications. This simplifies power distribution in many industrial settings.
8. No Electrolysis Issues: DC generators can produce electrolysis when used in certain applications, such as electrolysis cells or underwater applications, which can lead to equipment corrosion and other issues. AC generators do not have this problem.
9. Historical Adoption: AC power generation and distribution systems gained widespread adoption during the late 19th century, partly due to the pioneering work of inventors like Nikola Tesla and the support of figures like George Westinghouse. This historical momentum contributed to the dominance of AC systems in many parts of the world.
While AC generators offer numerous advantages, it’s important to note that DC generators still have specific applications where they excel. For example, DC generators are commonly used in applications like battery charging, electroplating, and certain industrial processes that require precise control of voltage and current.
Ultimately, the choice between AC and DC generators depends on the specific requirements of the application, including voltage needs, load types, and the availability of an existing electrical grid. Modern technology has also made it possible to convert between AC and DC power efficiently, allowing for the integration of both types of generators into diverse electrical systems.
Is an AC generator called an alternator?
Yes, An AC generator is called an alternator. Electrical AC generators are an electrical device that converts mechanical energy into alternating electrical energy.
A linear alternator or moving in the armature with the magnet’s stationary field is utilized in a variety of areas.
One of the main distinctions between generators and alternators is the fact that in an alternator, there is an armature that is stationary, and the field turns. In contrast, in the generator, the armature turns, and the field remains stationary.
Install my Free Android App on Google Play:
Electrical Cables Most Common Tables
And, my Electrical Calculations App “”
Discover more great content by subscribing to My channel
Looking to stay ahead of the game in the world of electrical engineering? Subscribe to my YouTube channel and gain access to exclusive content you won’t find anywhere else!
The staff I recommend
(Amazon Affiliate Links to products I believe are high quality):
- Economy 120 Volt/60Hz AC Power Source – Step-Down Voltage & Frequency Converters 1800W
- UNI-T Digital Multimeter Tester UT139C
- 50-Amp Extension Cord for RV “100ft”
- Voltage Stabilizer 110/220v
- Hair Dryer “best selling“
- TOSHIBA EM131A5C-BS Countertop Microwave Ovens
Disclaimer: This contains affiliate links to Amazon products. I may earn a commission for purchases made through these links.